International Year of Quantum and the Decade Ahead
An Editorial from Physical Review X Quantum Announcing the Launch of the IYQ Collection
In the summer of 1925, on the windswept island of Helgoland, a young Werner Heisenberg outlined matrix equations that would forever change our understanding of nature. Concurrent work by Erwin Schrödinger, who postulated a complementary wave-equation theory and showed its equivalence to Heisenberg’s matrix formalism, helped the scientific community to gradually embrace the counterintuitive concepts faced at the time. Together, these revolutionary principles became the cornerstone of quantum mechanics—a theory that, over the next century, would face relentless scrutiny [1] and ultimately serve as the foundation for technologies capable of manipulating single atoms and photons [2,3]. Today, as the world witnesses the development of quantum computers and grapples with their implications [4], UNESCO has declared 2025 the International Year of Quantum Science and Technology.
To celebrate this milestone, APS and the Physical Review journals reflect on their shared journey with quantum science—one of breathtaking discoveries and transformative ideas [5]. But what role does a young journal like PRX Quantum play in this momentous celebration?
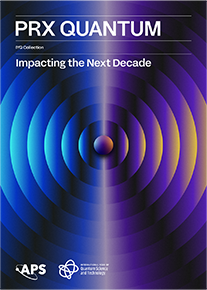
Any historian would argue that understanding the past is essential to shaping the future. At PRX Quantum, we constantly seek breakthroughs that redefine boundaries and open new frontiers. To honor 100 years of quantum mechanics, we present a special collection. This begins with a historical perspective [6] that explores the intricate dance between fundamental science and its technological offspring. Building on this perspective, we examined our recent publications and selected a handful of papers that offer a glimpse into the future of the field.
The path to realizing century-old thought experiments—once likened by Schrödinger to endeavors as silly as trying to raise Ichthyosauria in the zoo—required countless ingenious technical and conceptual breakthroughs. This fascinating journey is captured in Prof. Haroche’s captivating article [6], which highlights the central role lasers have played in quantum science.
As Prof. Haroche notes, we are now witnessing a renaissance of research on Rydberg atoms. Quantum computing with neutral atoms, prominently featured in our recent publications, is poised to significantly influence the field in the coming years. Remarkably, the laser’s offspring, optical tweezers [7,8], have emerged as a ubiquitous tool driving many breakthroughs in this arena. We highlight techniques to assemble atom-arrays [9], an architecture to effectively build a large-scale fault-tolerant quantum computer [10], and strategies to achieve record-high performances [11]. In concert, those results show a compelling path forward.
Superconducting qubits [12], a cornerstone of many quantum computing architectures, arose as an alternative system that drew heavily from the successes of cavity QED with atomic systems. They offer superior speed and practicality owing to their integration into standard microwave electronics. While transmons remain the dominant paradigm for superconducting qubits, there is growing interest in a related cousin, the fluxonium qubit. With its exceptional coherence and high anharmonicity, offering greater flexibility in circuit design, fluxonium holds significant promise. We anticipate exciting developments in this area [13,14].
The quantum landscape is vast, offering a playground of platforms and physical systems for exploring fundamental questions or pursuing specific applications. While it’s impossible to cover all such pathways, Prof. Haroche’s article inspired us to highlight the latest advances in integrated photonics [15], given the pivotal role of optics in quantum research. After all, photons enabled the violation of Bell’s inequalities, showcasing one of quantum mechanics’ most distinguishing features [16–18]. Likewise, optical cooling and trapping have led to some of the most striking demonstrations of quantum statistical principles, most notably the emergence of Bose-Einstein condensates [19,20]. We couldn’t resist offering a glance at the latest developments in controlled quantum chemistry with ultracold polar molecules [21].
In previous decades, the focus was on controlling individual quantum systems. Today’s challenges lie in managing interactions, scaling up system sizes, and verifying the status of large quantum systems or operations. Another trend observed in our journal is the development of theoretical tools for efficient tomography [22], and explorations on how to best bring together quantum processing and machine learning within formalized computer science theories [23].
Several fundamental questions remain about the key ingredients, and correct mix, needed to make a processor truly quantum—or, conversely, one that can be efficiently simulated classically [24]. Our journal reflects on the ongoing flurry of innovative algorithms, smart architectural choices, and hybrid techniques that steadily advance the overarching goal of fault-tolerant quantum computing. Adaptive quantum circuits is one such example. By leveraging mid-circuit measurements and feedforward, a promising approach shows how to efficiently prepare many-body entangled states even on low-depth near-term hardware [25].
Error correction plays a pivotal role in strengthening the quantum community’s confidence towards the feasibility of building a large-scale quantum machine [26,27]. Its history is just as fascinating as it was decisive in boosting worldwide investment in quantum science and technology. Research in this fast-paced area spans a vast spectrum, from highly mathematical and abstract code design to hardware-integrated and engineering-driven solutions. As a small glimpse into recent developments, we highlight three outstanding contributions: an ingenious implementation of the hallmark Steane code on ion traps [28]; a protocol that simplifies the implementation of low-density parity-check (LDPC) codes [29]—a resource-efficient alternative to surface codes; and a fundamental study that draws inspiration from topological error correction to deepen our understanding of phases of matter [30].
As has been long put forward by Shannon, key concepts in information theory are deeply connected with notions in thermodynamics, such as entropy. The connection between these fields—and the role of knowledge in thermodynamics—has a rich history [31], with a notable example being the resolution of Maxwell’s demon paradox [32]. At the same time, quantum mechanics is fundamentally a science of information. We couldn’t conclude this collection without highlighting the fascinating ideas emerging at the intersection of these disciplines. Recent advances in quantum thermodynamics further strengthen this connection, linking concepts from computational complexity to the study of the cost of thermal operations [33]. These costs have profound implications for quantum technologies [34] and are also tied to fundamental precision bounds, as demonstrated by a novel methodology that examines trade-offs in nonequilibrium Markovian open quantum systems [35].
Many discoveries arise from unexpected connections. We hope this curated collection sparks inspiration and insight—whether through the experimental and theoretical methods it showcases or the conceptual ideas it advances. This collection is but a snapshot, capturing some of the most compelling research published on our pages in recent months. The scope of PRX Quantum and quantum research extends far beyond what we could include here. The forest of quantum science consists of many trees and there is much fruit to be harvested in its varied branches, such as quantum sensors, metrology, and communications, which we leave for future spotlights. We eagerly anticipate the breakthroughs you will make in these and other areas, shaping the next decade of quantum science and technology.
References (35)
- Among multiple tests, the very linearity of Shrödinger’s equations has been experimentally verified as shown here: J. J. Bollinger, D. J. Heinzen, Wayne M. Itano, S. L. Gilbert, and D. J. Wineland, Test of the linearity of quantum mechanics by rf spectroscopy of the 9Be+ ground state, Phys. Rev. Lett. 63, 1031 (1989).
- Serge Haroche, Nobel lecture: Controlling photons in a box and exploring the quantum to classical boundary, Rev. Mod. Phys. 85, 1083 (2013).
- David J. Wineland, Nobel lecture: Superposition, entanglement, and raising Schrödinger’s cat, Rev. Mod. Phys. 85, 1103 (2013).
- Ivan H. Deutsch, Harnessing the power of the second quantum revolution, PRX Quantum 1, 020101 (2020).
- See a collection of Quantum Milestones published by Physics Magazine throughout 2025, and an upcoming collection on quantum foundations organized by the Physical Review journals.
- S. Haroche, Laser, offspring and powerful enabler of quantum science, PRX Quantum 6, 010102 (2025).
- A. Ashkin, Acceleration and trapping of particles by radiation pressure, Phys. Rev. Lett. 24, 156 (1970).
- A. Ashkin, J. M. Dziedzic, J. E. Bjorkholm, and Steven Chu, Observation of a single-beam gradient force optical trap for dielectric particles, Opt. Lett. 11, 288 (1986).
- M. A. Norcia, H. Kim, W. B. Cairncross, M. Stone, A. Ryou, M. Jaffe, M. O. Brown, K. Barnes, P. Battaglino, T. C. Bohdanowicz et al., Iterative Assembly of 171Yb Atom Arrays with Cavity-Enhanced Optical Lattices, PRX Quantum 5, 030316 (2024).
- Yiyi Li and Jeff D. Thompson, High-rate and high-fidelity modular interconnects between neutral atom quantum processors, PRX Quantum 5, 020363 (2024).
- R. B.-S. Tsai, X. Sun, A. L. Shaw, R. Finkelstein, and M. Endres, Benchmarking and fidelity response theory of high-fidelity Rydberg entangling gates, PRX Quantum 6, 010331 (2025).
- Jens Koch, Terri M. Yu, Jay Gambetta, A. A. Houck, D. I. Schuster, J. Majer, Alexandre Blais, M. H. Devoret, S. M. Girvin, and R. J. Schoelkopf, Charge-insensitive qubit design derived from the Cooper pair box, Phys. Rev. A 76, 042319 (2007).
- Helin Zhang, Chunyang Ding, D. K. Weiss, Ziwen Huang, Yuwei Ma, Charles Guinn, Sara Sussman, Sai Pavan Chitta, Danyang Chen, Andrew A. Houck, Jens Koch, and David I. Schuster, Tunable inductive coupler for high-fidelity gates between fluxonium qubits, PRX Quantum 5, 020326 (2024).
- Wei-Ju Lin, Hyunheung Cho, Yinqi Chen, Maxim G. Vavilov, Chen Wang, and Vladimir E. Manucharyan, 24 days-stable CNOT gate on fluxonium qubits with over 99.9% fidelity, PRX Quantum 6, 010349 (2025).
- Y. Pang, J. E. Castro, T. J. Steiner, L. Duan, N. Tagliavacche, M. Borghi, L. Thiel, N. Lewis, J. E. Bowers, M. Liscidini, and G. Moody, Versatile chip-scale platform for high-rate entanglement generation using an AlGaAs microresonator array, PRX Quantum 6, 010338 (2025).
- N. David Mermin, Is the Moon there when nobody looks? Reality and the quantum theory, Phys. Today 78, 28 (2025).
- Nicolas Brunner, Daniel Cavalcanti, Stefano Pironio, Valerio Scarani, and Stephanie Wehner, Bell nonlocality, Rev. Mod. Phys. 86, 419 (2014).
- Scientific Background on the Nobel Prize in Physics 2022, “FOR EXPERIMENTS WITH ENTANGLED PHOTONS, ESTABLISHING THE VIOLATION OF BELL INEQUALITIES AND PIONEERING QUANTUM INFORMATION SCIENCE” Advanced information. NobelPrize.org. Nobel Prize Outreach (2025). https://www.nobelprize.org/prizes/physics/2022/advanced-information/.
- E. A. Cornell and C. E. Wieman, Nobel lecture: Bose-Einstein condensation in a dilute gas, the first 70 years and some recent experiments, Rev. Mod. Phys. 74, 875 (2002).
- Wolfgang Ketterle, Nobel lecture: When atoms behave as waves: Bose-Einstein condensation and the atom laser, Rev. Mod. Phys. 74, 1131 (2002).
- S. Finelli, A. Ciamei, B. Restivo, M. Schemmer, A. Cosco, M. Inguscio, A. Trenkwalder, K. Zaremba-Kopczyk, M. Gronowski, M. Tomza, and M. Zaccanti, Ultracold LiCr: A new pathway to quantum gases of paramagnetic polar molecules, PRX Quantum 5, 020358 (2024).
- R. King, D. Gosset, R. Kothari, and R. Babbush, Triply efficient shadow tomography, PRX Quantum 6, 010336 (2025).
- Haimeng Zhao, Laura Lewis, Ishaan Kannan, Yihui Quek, Hsin-Yuan Huang, and Matthias C. Caro, Learning quantum states and unitaries of bounded gate complexity, PRX Quantum 5, 040306 (2024).
- Yifan Zhang, and Yuxuan Zhang, Classical simulability of quantum circuits with shallow magic depth, PRX Quantum 6, 010337 (2025).
- Kevin C. Smith, Abid Khan, Bryan K. Clark, S. M. Girvin, and Tzu-Chieh Wei, Constant-depth preparation of matrix product states with adaptive quantum circuits, PRX Quantum 5, 030344 (2024).
- Peter W. Shor, Scheme for reducing decoherence in quantum computer memory, Phys. Rev. A 52, R2493 (1995).
- Andrew Steane, Multiple-particle interference and quantum error correction, Proc. R. Soc. Lond. A 452, 2551 (1996).
- Lukas Postler, Friederike Butt, Ivan Pogorelov, Christian D. Marciniak, Sascha Heußen, Rainer Blatt, Philipp Schindler, Manuel Rispler, Markus Müller, and Thomas Monz, Demonstration of fault-tolerant steane quantum error correction, PRX Quantum 5, 030326 (2024).
- Noah Berthusen, Dhruv Devulapalli, Eddie Schoute, Andrew M. Childs, Michael J. Gullans, Alexey V. Gorshkov, and Daniel Gottesman, Toward a 2D local implementation of quantum low-density parity-check codes, PRX Quantum 6, 010306 (2025).
- Yaodong Li, Nicholas O’Dea, and Vedika Khemani, Perturbative stability and error-correction thresholds of quantum codes, PRX Quantum 6, 010327 (2025).
- A. Bérut, A. Arakelyan, A. Petrosyan, Sergio Ciliberto, Raoul Dillenschneider, and Eric Lutz, Experimental verification of Landauer’s principle linking information and thermodynamics, Nature 483, 187 (2012).
- Koji Maruyama, Franco Nori, and Vlatko Vedral, Colloquium: The physics of Maxwell’s demon and information, Rev. Mod. Phys. 81, 1 (2009).
- A. Munson, N. B. T. Kothakonda, J. Haferkamp, N. Yunger Halpern, J. Eisert, and P. Faist, Complexity-constrained quantum thermodynamics, PRX Quantum 6, 010346 (2025).
- Alexia Auffèves, Quantum technologies need a quantum energy initiative, PRX Quantum 3, 020101 (2022).
- Tan Van Vu, Fundamental bounds on precision and response for quantum trajectory observables, PRX Quantum 6, 010343 (2025).
This piece was posted on the APS Physical Review Journals website.
For general questions about IYQ, please contact info@quantum2025.org. For press inquiries, contact iyq2025@hkamarcom.com.